Surface Reactions and Film Formation
In order for a film to grow, some process must convert atoms or molecules in the gas phase into atoms or molecules at the surface of the film, which can then be incorporated into it. This process is normally thought of as taking place in several steps. First, a molecule "adsorbs" on the surface (gets stuck on it). Adsorption is often divided into physisorbtion (where the adhesive force is essentially the van der Waals interaction) and chemisorption (where much stronger covalent bonds are formed between the molecule and the surface). Once molecules are on the surface, they may wander around a bit and perhaps react with some other surface species, releasing products and forming film. The mobility of surface species is largest on metallic and semiconducting surfaces, where bonding is not very directional, and rather limited on dielectrics where highly directional covalent bonds tend to hold a molecule in one place once it is chemisorbed.
Surfaces are potentially complex beasts. The creation of the surface from a continuous bulk material results in something like one broken ("dangling") bond for each surface atom. Such a circumstance would lead to a very high energy, so typically surfaces try to reduce their energy either by adsorbing atoms or molecules from the ambient, or by reconstructing: changing the arrangement of atoms at the surface to allow additional bonding of the surface atoms. Real surfaces often involve both influences; a "clean" single-crystal silicon surface created by wet etching in dilute HF is actually terminated mostly in Si-H, with some Si-F present at steps and other defects in the surface.
A great deal of surface research has been done in the last 40 years on the actual interactions of atoms and molecules with surfaces: exactly where adsorbed atoms like to sit, what sorts of steps and defects are most reactive, what the actual mechanisms of reaction are. Unfortunately, most of this research is done in ultra-high vacuum on nearly ideal surfaces, and is thus not directly relevant to most CVD, which takes place at pressures from a few mTorr to an atmosphere. In this tutorial we shall be content to provide the very simple conceptual framework in which most of the empirical deposition research has been done: we treat all surface sites as identical and absorb actual behavior into a few experimental coefficients.
Adsorption Isotherms
The first question we'll try to address is to establish a plausible form for the relationship between the concentration of a species in the gas phase, and the amount of species adsorbed on a surface. Our simple view of the surface asserts that there are a fixed total number of reactive sites upon which an incoming atom (adatom) or molecule can adsorb. Once a site is occupied, a second molecule will not adsorb on it. [This is equivalent to the assumption that the precursor does not condense on the surface.]
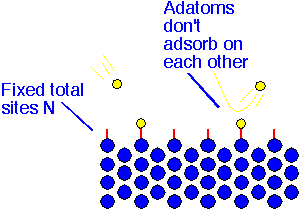
The "coverage" is the ratio of occupied sites to total sites. The steady-state value results when the number of atoms adsorbing is equal to those desorbing, if no reaction is taking place.

The number of atoms or molecules adsorbing is proportional to the concentration in the gas phase, or equivalently to the partial pressure P, and to the number of vacant sites -- that is, to N*(1-coverage). The number desorbing is proportional to the number of filled sites. In steady state these two contributions balance.

We can solve for the coverage as a function of the gas phase concentration, obtaining the expression shown below: the Langmuir isotherm. This is known as an isotherm since it describes adsorption at a fixed temperature. In general, with increasing temperature desorption is favored, and the saturation coverage decreases.
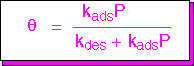
This expression describes archetypal surface behavior: at low concentrations, the surface coverage is proportional to the gas phase concentrations, whereas at high concentrations coverage is saturated and no longer dependent on the gas phase.
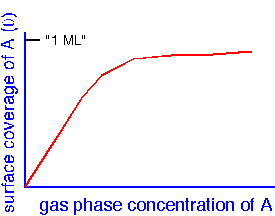
Surface Reaction Rate
The simplest treatment of surface chemistry is based on the derivation of the Langmuir adsorption isotherm: multiple species compete for the available surface sites, and once adsorbed may move on the surface until they either desorb or react. By a very similar derivation we can obtain an expression of the form:
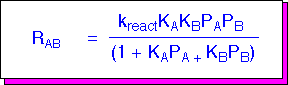
Such an expression is known as Langmuir-Hinshelwood kinetics. Physically, what is says is that the two constituents compete for adsorption sites, and react with each other. For any given set of values of the reaction rate coefficients, the net reaction rate will be maximized at a specific set of values of the partial pressures of A and B. "Too much" of either reactant will saturate the surface sites and lead to a decrease in reaction rate. If the reaction in question results in the creation of film, then this reaction rate may also be the deposition rate. A deposition rate displaying a maximum in the concentration of reactants might indicate that the rate is controlled by adsorption/desorption processes (but have a care! gas phase reactions and transport limitations can produce similar behavior).
Step Coverage, Gap Fill, Conformality
A process described by the sort of Langmuir adsorption we've discussed above suggests that the likelihood that an incoming molecule will adhere to a surface -- it's Reactive Sticking Coefficient, RSC -- is likely to depend on the conditions on the surface as well as on the characteristics of the incoming molecule. A radical or other unstable precursor molecule is likely to react readily with a surface site and stick wherever it strikes. A more stable precursor may not react readily, particularly at higher temperatures or when the surface is already saturated with adsorbents.
The likelihood of reaction is important because successive adsorption and desorption is one of the key mechanisms determining the effect of topography of a surface on the thickness of a film deposited on that surface. The ability of a film to cover a step or fill a gap or hole is often of critical importance in semiconductor device manufacturing, and is one of the key advantages of chemical vapor deposition over evaporation or sputtering of thin films.
Let's imagine, for example, that we have a couple of metal lines (e.g. aluminum) on a substrate, that are being used as wires. We need to cover them up with an insulating dielectric, so that a subsequent layer of metal may be deposited without the two sets of metal touching each other and shorting the lines out. The desired result is depicted in cross-section at left: the film should completely coat the metal features, and fill the gaps between them.
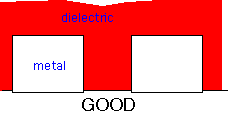
Unfortunately, it is often the case that deposited films grow more readily on horizontal, exposed surfaces, than within trenches or holes, resulting in poor coverage of the surface with the film. The gaps that are formed can lead to shorts, reliability problems, etching failures, and other generally undesirable phenomena.
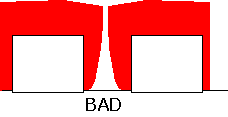
Particularly in dielectric deposition, it has been shown that an important mechanism for transport of the material into gaps and holes is through multiple adsorption and re-emission from the surface: that is, from precursors having a low RSC. When such "reflections" occur many times, the flux of precursor to the surface inside a hole may differ by very little from the flux onto the horizontal surfaces.
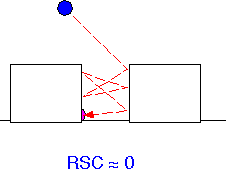
When RSC is large, the flux to any location on a surface is essentially determined by the solid angle of gas that would be "visible" to an observer looking up from that location. An observer at the bottom of a deep trench, like a pedestrian in downtown San Francisco [or pick your own favorite city!], can't see much when looking up. Thus the flux and growth rate are high on the surfaces but low in a trench, and conformality of the resulting film is poor (here assuming that the adsorbed species don’t move around much, often true for dielectrics, less so for e.g. high-temperature epitaxial growth).
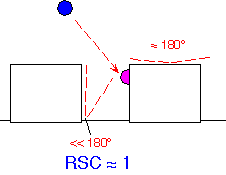
To the extent that RSC is determined by adsorption kinetics of the sort we discussed in connection with the Langmuir isotherm, conformality is likely to be improved if the surface is saturated: that is, by the use of high concentrations of the reactants to reduce the likelihood that any given molecule will find a reactive site on the surface. However, it is often difficult to arbitrarily increase precursor concentration: parasitic gas phase reactions may occur, or the quality of the film may decrease when the growth rate is high.
Conformality can also provide key clues to chemistry. For example, in deposition of silicon dioxide from TEOS (tetra ethoxy silicate, Si(OC2H5)4) and oxygen, McVittie and coworkers have shown that one must assume one precursor with a fairly high value of RSC (e.g. around 0.3) to explain the observed deposition profiles. As we discussed in our introduction, the flux of molecules to a surface even at LPCVD pressures greatly exceeds observed deposition rates. We can conclude that the precursor to deposition must be present in very low concentrations, much lower than the input concentration of silane: that is, a gas phase reaction must be occurring to produce a relatively reactive molecule that is actually responsible for deposition.
reference: "Two precursor model for low-pressure chemical vapor deposition of silicon dioxide from tetraethylorthosilicate" M. IslamRaja, C. Chang, J. McVittie, M. Cappelli, and K. Saraswat [Stanford University] J. Vac. Sci. Technol. B11 720 (1993)
An Intriguing Connection: Conformality and Enthalpy
The reaction kinetics at a surface are complex and specific to the exact surface composition and configuration, as well as the nature of the reacting species. It isn't obvious that equilibrium quantities should be informative about surface phenomena, but a quick look at the data suggests that a connection may exist. The table below shows some important overall CVD reactions, along with a general classification of the resulting film conformality.
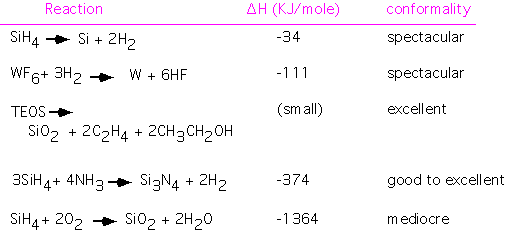
[Note that we have considered the first step of the TEOS reaction only -- we have assumed that the subsequent oxidation of the organic ligands, ethylene and ethanol here, may release a lot of energy but plays little role in the deposition.]
We see a general trend that good conformality is associated with reactions with small standard free energy change, whereas large energy releases produce poorer step coverage. The obvious speculation is that larger energy releases tend to favor the formation of reactive intermediate species, with high sticking coefficient on most surfaces.
Return to Tutorial Table of Contents
Book version of the CVD Tutorial