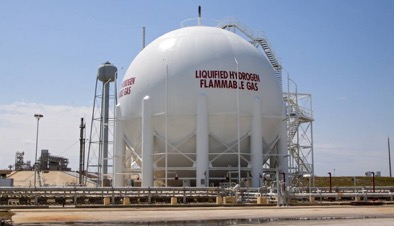
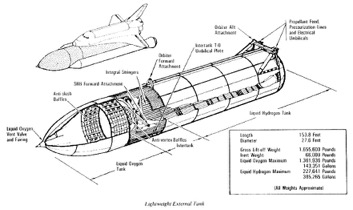
Figure 1: a NASA hydrogen storage tank, and the space shuttle external tank that the hydrogen is stored for.
When you're not quite as far up, it's a bit harder. Commercial aviation is based on aviation fuels, modest variants of gasoline. The effective energy density of these fuels is not that great. For example, a propeller-driven Cessna Skylane 4-seat airplane in cruise delivers about 11 or 12 MJ/kg from fuel that has over 40 MJ/kg in it. Commercial airliners like the 737-800 achieve similar values. Liquid hydrogen takes a bit of additional mass for the carefully-insulated storage tank and plumbing, but overall system mass efficiency around 60% is achievable [1]. That gives us an effective density of about 143(0.6) = 86 MJ/kg, still loads better than aviation fuel. We can burn the hydrogen in existing turbojet engines, with a slight degradation in efficiency vs. jet fuel [2]. It's very likely that minor modifications would provide comparable efficiency to that of the jet fuel. Or we can use fuel cells to convert the hydrogen to electricity. If we achieve 60% efficiency from the fuel cells, and then use the electricity in 90%-efficient electric motors, driving propellers or turbofans with (say) 80% propulsive efficiency, we would expect to get effective energy density of around 37 MJ/kg, much better than a conventional aircraft achieves.
Recall, though, that the volume density of even liquid hydrogen is mediocre; the system doesn't weigh as much as a conventional aviation fuel system for the same energy content, but it takes more space. Figure 2 shows a simplified example of what you'd need to convert a conventional airliner:
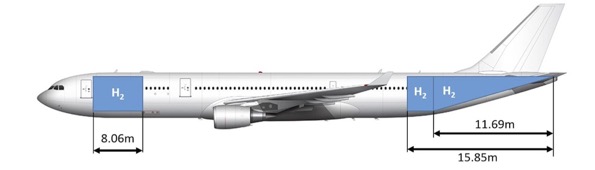
Figure 2: a rough estimate of what's needed to convert a conventional jet aircraft to liquid hydrogen [3].
For a commercial airliner, space is passengers (although from the passenger's viewpoint it often seems no space is allocated anyway). It also takes on the order of 30 MJ/kg to convert gaseous hydrogen to liquid hydrogen [4], so it's wasteful and costly. In principle you can get some of that energy back when you warm the stuff up, but this is not commonly attempted. And liquid hydrogen requires a well-known but complex and expensive infrastructure to make, ship, store, and use.
So it's plausible that airliners using liquid hydrogen would actually benefit in performance — but they'd need significant redesign to avoid trading passengers for liquid hydrogen tanks. And you need all your takeoff and landing locations to support refueling. You can't make use of any infrastructure until all (or at least most of) the infrastructure is built. So who will build an airplane that can't fly anywhere? And without an airplane who would build a fueling infrastructure? Talk about domain walls!
An alternative path is to make aviation fuel, or its equivalent, using means that don't generate net carbon dioxide. For example, you can make fuel from plants — biofuel — that is formed by capturing carbon dioxide from the air. This is easy to sell as ecologically beneficial, and can be profitable for the people growing the plants. Some of the crops employed, such as Jatropha and Camelina, are not normally used as food crops and can be grown in regions that are not prime land for food, or as a rotation crop in food-growing regions [5]. These sources are generally free of sulphur compounds and thus don't emit sulfur dioxide when burnt. But depending on how growth is done, it can lead to more carbon dioxide release than it saves — this being the case if existing woodlands are cut down to produce the requisite crops [6].
So in the near term we'd expect the aircraft and airports to remain almost unchanged, but with part of the jet fuel supply coming from biological sources. At the present time five airports in the world provide biofuel mixes. The current cost of biofuels is modestly more than aviation fuel (or it was until the pandemic crashed the cost of everything — but that is presumably a temporary if painful condition for the world). Investment in growth or synthesis and refining is needed, but the magnitude is comparable to ordinary investment in fuel processing. But the overall efficiency of the process isn't very high — typically about 0.3 to 0.5% of the sunlight incident is converted into useful fuel, compared to photovoltaic efficiency at least 20 times higher, and that doesn't account for the energy used in refining. At full scale this devotes a lot more land to energy production than would be the case using photovoltaic panels and electrolysis. The use of algae is significantly more efficient than large plants for this purpose, but the required technology is still under development. So it's a bit unclear if this is the best long-term option for replacing fossil fuel.
It's interesting to speculate on a path through which hydrogen-powered flight would replace aviation fuel. Just as in the case of land transport, this path goes sideways. In the last two or three years, on the order of a billion dollars has been invested in companies working on various forms of electric aviation. A few of these companies — Alaka'I, Zeroavia, and HES Energy Systems — are designing hydrogen-powered planes today. Others — Bye Aerospace, Eviation, Jaunt Air Mobility, Joby Aviation, Kitty Hawk, Skylax for example — are targeting battery power at present [7].
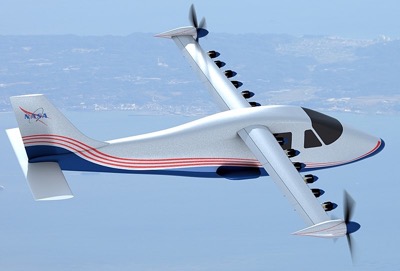
Figure 3: a NASA X-57 aircraft using Joby electric engines (Wikipedia).
A commercially successful implementation of any of these aircraft would create a market for electrically-powered aircraft. And as soon as you have a battery-powered airplane performing a function, you'll wish you had more energy at the same weight. The same problem already exists in the very substantial market for small electric unmanned aerial vehicles (UAV's = drones). So far, the volume/price domain wall, and the mediocre energy density of compressed-hydrogen systems, have blocked widespread implementation of hydrogen storage in this market as well. If either market makes the transition, that could provide a hydrogen-powered aerial infrastructure that then becomes available for the large electric aircraft that have been extensively explored but not implemented [8].